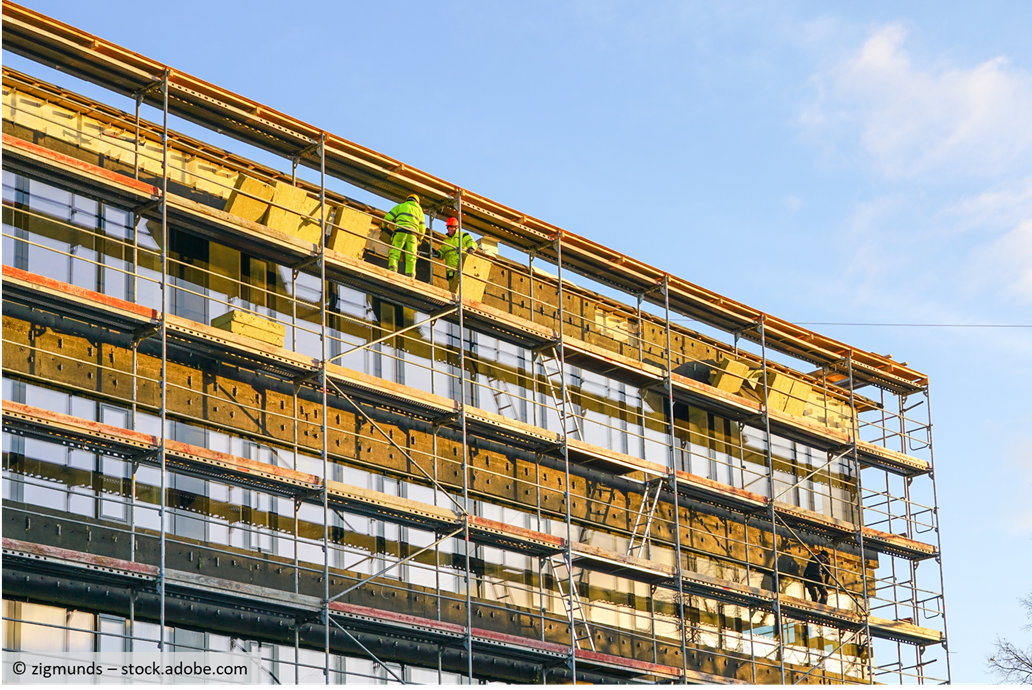
Why we should change the way we environmentally assess building retrofits
On 09.05.2022 by Linus WalkerBy Linus Walker
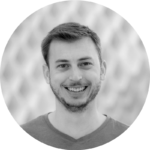
Linus Walker is a doctoral researcher at the Chair of Architecture and Building Systems. In his research, he focuses robust performance of building systems. This includes the simulation-based assessment of building system performance under different future scenarios. He is further involved in teaching activities on energy and climate systems and building-integrated photovoltaics. Before starting his doctorate studies, Linus completed a Master’s degree in Energy Science and Technology at ETH Zürich.
Building retrofits are increasingly assessed from an environmental perspective. Standard assessment methods, however, largely do not consider future developments and fail to take into account the state of climate emergency where the time horizon is substantially shorter than the expected building lifetime. Therefore a more forward-looking analysis is required. Including global warming, electricity decarbonization, and aligning the analysis period with 2050 goals give greater weight to material-based embodied emissions. I, therefore, claim that construction materials are substantially more relevant in the fight against global warming than currently considered in most assessments.
Building retrofits have been previously discussed on the ETH Energy Blog. It was shown why building retrofits are important, that tenants are willing to pay more rent in retrofitted buildings, and how modern, interconnected buildings can become more efficient thanks to big data and advanced algorithms. Further, it was discussed that we need innovative policies to reach building stock decarbonization goals.
This time, I want to talk about the methods used to assess building renovations’ environmental impact, specifically their impact on climate. Most of the discussion points are valid for assessing both new constructions and retrofits.
From the seventies to the early 2000s, a building using less energy per square meter was considered more sustainable. The energy use per square meter of floor area was the primary indicator. Regulations targeted building envelope measures such as increased insulation or window replacement to reduce energy consumption. As presented in Figure 1 below, this resulted in lower heating demands for newly built and retrofitted buildings. When focusing on energy demand, the heating system is only of secondary importance. Substituting oil and gas heatings with non-fossil heatings was therefore not incentivized.
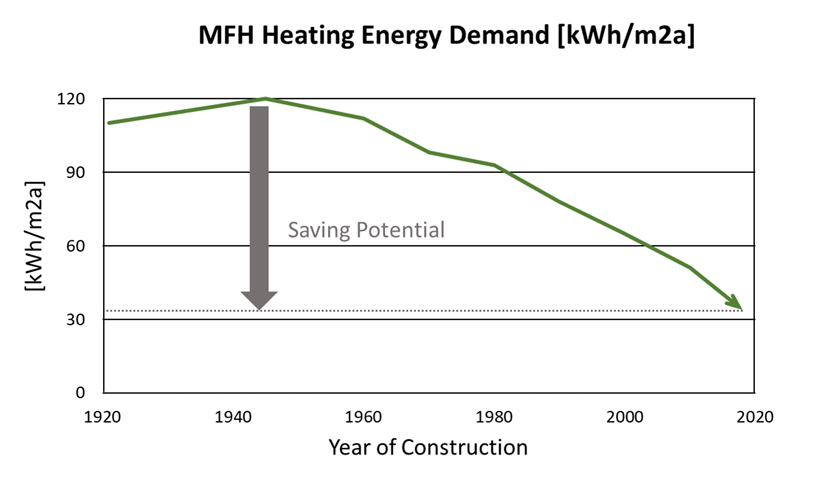
Figure 1: Average specific energy demand for Swiss multifamily houses according to their construction years. [Data from Streicher et al.] A good retrofit of a building from the 1970s could save up to 70% of operational heating energy demand.
After the awareness of global warming increased in the 2000s, calculating the GHG emissions of heating systems became more common. However, as buildings got more efficient, the emissions from materials started becoming more critical. Nowadays, environmental assessment is increasingly based on life cycle assessment (LCA). LCA defines the impact of a product or a service over its lifetime for multiple impact categories. Building specific methodologies are defined in national and international standards (e.g., SIA, SNBS, EPBD, EN15804) that sometimes rely only on parts of an LCA or even go beyond LCA and include, for example, social criteria. Here I will focus on calculating GHG emissions, which is done similarly in most standards. Typically embodied and operational emissions are distinguished and ultimately summed up to assess the whole impact (See Figure 2).
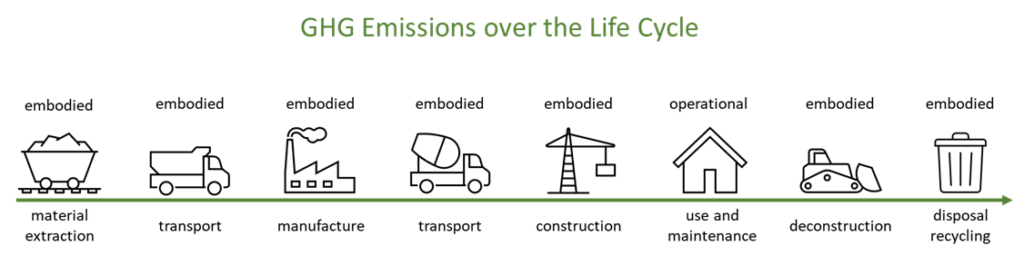
Figure 2: Emissions related to material production, construction, installation, and disposal are included in embodied emissions, while the energy consumption-related emissions are considered operational.
1. Static assumptions
Average annual operational emissions are considered to be constant over the entire life cycle. They are calculated based on a typical year and then extrapolated as shown in Figure 3 on the left. Similarly, it is assumed that components are replaced with identical components at their end of life. That means that very likely developments that we must expect to happen in the foreseeable future are not considered.
For example, multiple research studies expect decreasing heating demand due to global warming. Less heating translates into less operational emissions. Furthermore, electricity-based systems (e.g., heat pumps) cause their operational impact via the GHG emissions of the electricity mix. There are many national and international goals to decarbonize the electricity sector. The electricity mix and the related GHG impact factor of electrical energy will therefore decrease over the following decades.
To be able to consider such changes in the environmental assessment of building retrofits, a more dynamic modeling approach is necessary.
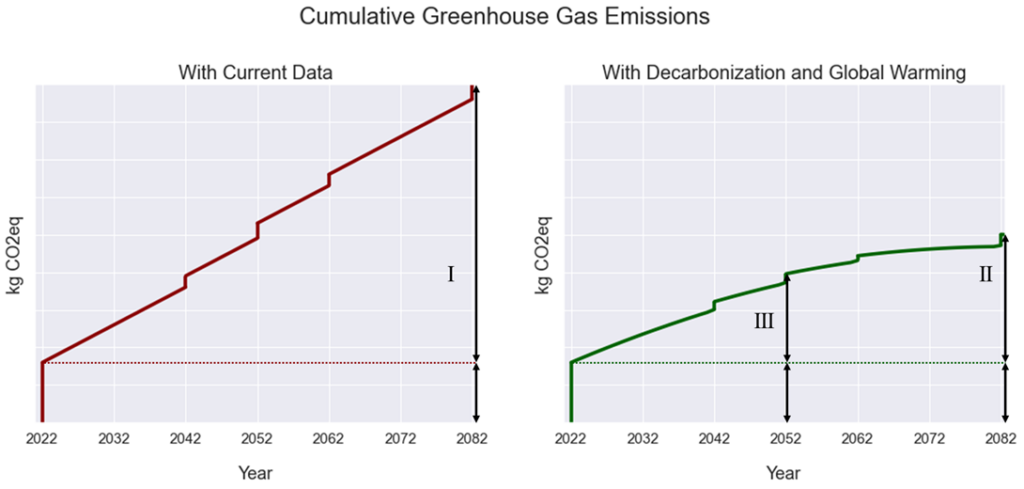
Figure 3: Cumulative emissions of a building retrofit (materials + operation). The bottom part indicated with the dashed line represents the embodied emissions from the construction that occur in the first year. L: Static assumptions on operation and replacement, R: Considering electricity grid and material decarbonization over time and effects of global warming. (Qualitative Assessment)
2. Long analysis period
In Swiss standards, the analysis period is 60 years, as this is the assumed lifetime of a building. If we, however, assume that we have ten years to reduce GHG emissions significantly and 30 years to net zero (see, e.g., IPCC), 60 years are no longer appropriate. This becomes even more apparent when we look at the cumulative emission curves in Figure 3. The long analysis period allows diluting emissions from long-living components despite the fact that all of the emissions from the production are happening now, using up our remaining carbon budget. In retrofits, typical long-living components are insulation materials or structural elements. Both usually come with high embodied emissions.
We can now reconsider the analysis by looking at Figure 3 again. In the standard assessment, we would compare buildings based on the results at point (I). If we include expected global warming and electricity decarbonization, we end up at point (II), drastically increasing the relative importance of the construction materials. If we also shorten the analysis period to 30 years, this effect becomes even stronger (see point III). Looking at the relative contribution of embodied emissions for the case shown below, we see a shift from below 20% (point I) to almost 40% (point III).
An additional point to raise connected to the long analysis period is that predictions are always connected to uncertainty. The further we plan ahead, the bigger the uncertainties usually get. For example, if we go back 60 years in the past, there weren’t any nuclear power plants in Switzerland. Now the first one is being dismantled, and current legislation does not allow the construction of new ones. How should anyone have been able to include that in an analysis in 1962? Although there is a whole field of research focusing on decision-making under deep uncertainty, in my opinion, this is another reason to question such a long analysis period.
Independent of the uncertainties, from this rather qualitative analysis, it becomes apparent that the embodied emissions of the initial construction or retrofit measure are generally undervalued and deserve more attention.
In my research, I did a quantitative assessment where I compared different retrofit measures under different methodological assumptions. Next to the above-mentioned aspects, I also looked at allocation methods of emissions related to PV electricity exported to the grid and carbon stored in biogenic materials. The results showed that the choice of assumptions can be decisive for retrofit strategy recommendations. For example, materials with high embodied emissions such as some insulation materials or window types can get less attractive from a GHG emissions point of view, which calls for more innovation in low-emission materials and products.
In conclusion, construction materials and their respective embodied emissions should get higher priority in the environmental assessment of buildings’ retrofitting measures. I urge policymakers and experts involved in establishing standards to do their best to align methodological assumptions with society’s decarbonization goals while incorporating appropriate assumptions along with uncertainty analysis methods. While policymakers can do their share by adapting construction standards, it is no less important that architects and engineers know about the burden they are placing on future generations by choosing construction materials poorly and that they consider this in their design decisions.
Cover photo by AdobeStock
Keep up with the Energy Blog @ ETH Zurich on Twitter @eth_energy_blog.
Suggested citation: Walker, Linus. “Why we should change the way we environmentally asses building retrofits”, Energy Blog @ ETH Zurich, ETH Zurich, May 9, 2022, https://blogs.ethz.ch/energy/building-retrofits/
If long-term assumptions about future energy sources can be misleading because energy policy can change, you should use the best solution at the moment, the best materials currently in terms of GHG emissions.
On the other hand, this can also lead to future problems. More heat pumps, for example, will lead to more winter electricity demand, and Switzerland is already importing electricity in winter.
Thank you for your comment. Indeed, the analysis is focused on GHG emissions and does not evaluate peak demand or winter demand. This could be modeled for example with a dynamic grid impact factor taking into account the GHG intensity of imports in a higher temporal resolution than currently done.