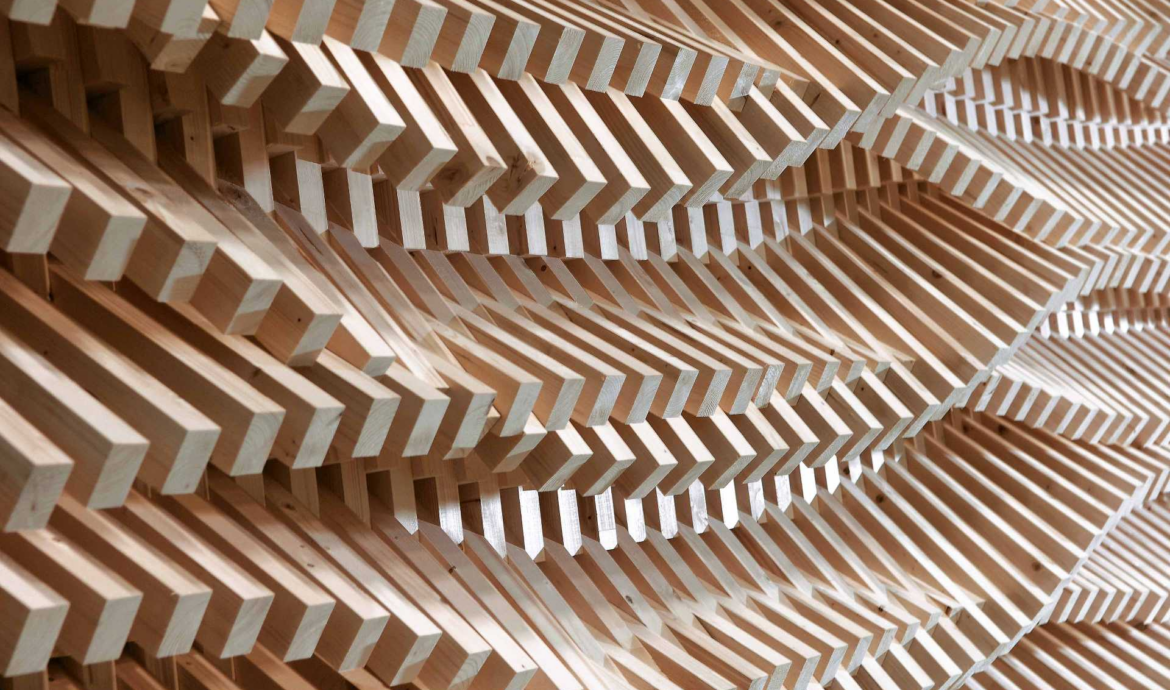
Wooden buildings: a natural carbon sink boosted by modern manufacturing technologies
On 23.06.2021 by Xuqian YanBy Xuqian Yan and Hao Wu
Xuqian Yan is a PhD student in energy informatics at Siemens Energy and the University of Oldenburg. She previously worked at the Energy Science Center at ETH Zurich to develop the Nexus-e energy system modeling platform. Her passion and ambition are to push forward the energy transition with digitalization.
Hao Wu is a master graduate from structural engineering at ETH. He is now working as a computational design engineer at Jan Knippers Ingenieure and has specific focus on the research and design of novel fibre and wooden structures.
Wood is an ancient construction material, but it has been gradually substituted with concrete and steel. However, the advantages of wood are now being (re)discovered as sustainability becomes a global interest and advanced manufacturing technologies become available. This article focuses on its advantages in carbon capture, digital construction, and prefabrication.
Wood, a natural carbon sink
Under the pressing climate crisis, numerous countries have pledged carbon neutrality. For example, Switzerland targets on achieving “net-zero” by 2050; China aims to “have CO2 emissions peak before 2030 and achieve carbon neutrality before 2060”; and the USA aims at 50-52% reduction in greenhouse gas from 2005 levels in 2030 and achieving net-zero by no later than 2050. Can wood, a natural carbon sink, contribute to realizing carbon neutrality?
The following figure answers this question: Yes, thanks to photosynthesis at its growth phase. The figure compares the life-cycle carbon sequestration/emission of cementitious (yellow line) vs. bio-based (blue line) materials. It can be seen that the cementitious materials emit more carbon during the whole lifecycle despite a slight decrease due to carbonation, while bio-based materials contribute to carbon sequestration in most of their life cycle because of photosynthesis at their growth phase.
Figure 1: Carbon sequestration/emission of cementitious and bio-based materials throughout the life cycle. At the end-of-life stage, the dashed lines for bio-based materials cover possibilities from instant oxidation for energy recovery, to re-use, recycling, or landfill scenarios. (Source)
However, as shown by the dashed lines in Figure 1, it is unclear whether bio-based materials can keep their advantage considering various end-of-life scenarios, for example:
- directly incinerated for energy recovery: in this way carbon stored in woods is instantaneously released but burning fossil fuels can be avoided;
- recycled for subsequent product cycles (i.e., multiple cascading): environmental impact is less than direct incineration;
- landfill: although landfilling can be viewed as a long-term carbon storage, it has higher greenhouse gas emissions overall than incineration or recycling; landfilling waste wood is prohibited in many parts of the European Union.
Among the listed scenarios above, direct incineration releases the carbon in wood instantaneously and therefore has the highest short term emission. This study examines this scenario and shows that with direct incineration for energy recovery (without carbon capture – this will be mentioned later) woods have less emission than concrete because of the substitution effect: generating energy from waste wood avoids the use of fossil fuels. Table 1 summarizes the life-cycle carbon emission of the base case in the study. It can be seen that concrete-framed buildings emit 36.6 tonnes of carbon (tC) over the life-cycle while wood-framed buildings avoids 24.1 tC emission. Note the negative values in the table are a combination of carbon removed from the atmosphere and avoided fossil fuel emissions.
Table 1: Life cycle carbon emissions [tC] of two functionally equivalent buildings (4-storey building with a usable floor area of 1190 m2): with structural frames of either concrete or wood (Source)
One step forward, the study shown above didn’t consider using CCS (carbon capture and storage) to capture the carbon released during the end-of-life energy recovery process. This leads to the re-release of the carbon stored in the woods. If instead, CCS is used to avoid the carbon emission during the energy recovery from woods (i.e., bioenergy with carbon capture and storage, BECCS), then another 12.1 tC can be eliminated by the wood-framed building, making the total carbon emission difference 72.8 tC.
Figure 2 shows an exemplary BECCS plant: Drax biomass power plant in UK. This plant incinerates biomass (including waste woods) to produce electricity. This process generates flue gas containing CO2, which is then captured and permanently stored in a geological storage under the North Sea.
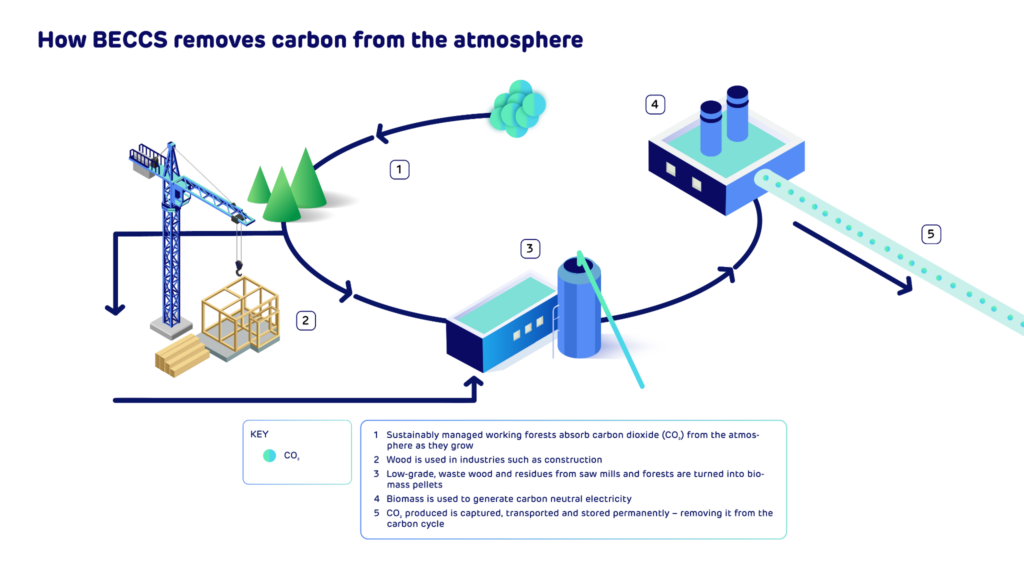
Figure 2: BECCS process in the Drax biomass power plant in UK
Boosters of wooden buildings: digital construction and prefabrication
The carbon-absorbing wooden structure is expanding its share in the building sector, as its unique characteristics meet the needs and trends of digital construction and prefabrication.
The construction field is embracing a digitalized era: besides sustainable, lightweight, multifunctional, and aesthetically pleasing, the construction material should also be receptive to parametrically driven forms and digital fabrication.
In this regard, wood is a manufacturing-friendly material for robots. Doubly curved beam/shell elements, panels of various shapes, diverse surface texture and complex joints can be precisely manufactured through digital and robotic fabrication with high efficiency. Intricate joint details, which used to be one of the hurdles for wood toward fast mass production, now become a source of its compelling charm.
Figure 3 shows the BUGA Wood Pavilion (University of Stuttgart ICD/ITKE) and its panel fabrication by robots. The oldest natural building biomaterial and the most cutting-edge robots, are very well matched with each other in the digital fabrication process.
(a) North view of BUGA Wood Pavilion
(b) Panel fabrication by two robots
Figure 3: BUGA Wood Pavilion as a demonstrator of computationally designed and digitally fabricated wooden building (Source: University of Stuttgart ICD/ITKE)
Prefabricated buildings are also becoming an increasingly popular choice when designing multi-family and commercial buildings, and wood is a particularly beneficial material for prefabrication. In Figure 4, the new Swatch headquarter in Biel is being assembled using prefabricated wooden elements.
Figure 4:The new Swatch headquarter in Biel is being assembled using prefabricated wooden elements. (Source: Dezeen)
Prefabricated buildings can help to solve many design, engineering and management challenges such as (Source):
- Higher material utilization through material cutting optimization and component reuse.
- Higher process efficiency by shortening on-site construction time and optimizing supply chain.
- Better quality control since most fabrication processes are conducted in a factory under controlled environment.
Prefabricated wood components are frequently used in light wood frame (beam, column, etc.) and mass timber (floor, wall, etc.) construction. In prefabricated buildings, wood is particularly beneficial (source), because:
- It is flexible in form-shaping.
- Small manufacturing tolerances and high degree of prefabrication can be achieved.
- Low self-weight allows for smaller cranes and foundations.
- It possesses multifunctionality: structural component, architectural element, decoration, etc.
- Easy demolition and re-assembly encourage the reuse of components.
However …
Some words of caution: Although wood emits less carbon than concrete over its lifetime, the emission difference between wood and steel/concrete will decrease as energy systems and industry processes continue to decarbonize. Also, we need to be careful of promoting plantation forests for wood supply because monoculture plantations may cause ecological damages.
Despite these considerations, the advantages of wooden structure in this era with the pressing climate crisis and advanced technologies are not to be ignored. First, wood can contribute to carbon capture by storing carbon within the material over its long-lasting life cycle, and it provides the opportunity to permanently store the carbon if the waste wood is handled with CCS. Besides, wood is well suited for robotic construction and its genes for prefabrication can be easily unfolded when combined with the right technology.
Cover picture is provided by ©Gramazio & Kohler, ETH Zurich. Michael Bühler, David Dalsass, Simon Filler, Roman Kallweit, Jonathan Roider (retrieved from https://magaceen.com/en/innovation/digital-building/)
Keep up with the Energy Blog @ ETH Zurich on Twitter @eth_energy_blog.
Suggested citation: Yan, Xuqian and Wu, Hao. “Wooden buildings: a natural carbon sink boosted by modern manufacturing technologies”, Energy Blog @ ETH Zurich, ETH Zurich, June 23, 2021, https://blogs.ethz.ch/energy/wooden-buildings/
Yes, wood use together with CO2 capture and storage at the end of the life cycle could be a viable solution for CO2 neutrality in an already largely decarbonised world. Even if humankind stopped using oil, gas and coal in 2050, there will still be around 1 ton of CO2 emissions per capita due to the remaining emissions from cement, steel and agriculture. In Switzerland, the annual wood harvest is 5 million tons, which corresponds to 8 million tons of CO2 or 1 ton of CO2 per Swiss citizen. If you sequester this wood (used e.g. for buildings) at the end of life, you would offset the Swiss emissions of 2050 from cement, steel and agriculture.
Advantage: seems to be be quite a natural kind of CO2 compensation.
Many thanks for the comment! The numbers comparing the annual wood harvest and hard-to-avoid emission indeed look very promising. This reminds me of a previous blog article https://blogs.ethz.ch/energy/climate-day/ where the importance of CCS to compensate hard-to-avoid emission is illustrated using a diagram from the Energy Perspectives 2050+.
How about gluelam? Since a lot of the modern technologies use glued timber, how does gluelam contribute as carbon sink with regard to re-use and the circular economy? Does gluelam really contribute to the carbon sink in the long run?
Modern technologies using glued timber:
https://www.ts3.biz/de/referenzprojekte/referenzen/2540-Fasanenhof.php
https://fagussuisse.ch/angebot/produkte/?prod=produkt-3
Modern technologies without glued timber:
https://www.naegeli-holzbau.ch/appenzellerholz.html#vollholz-elementbau
https://www.thoma.at/?lang=en
https://www.sohm-holzbau.ch/diagonalduebelholz
Many thanks for your comment!
Glued laminated timber is indeed a popular construction material. The CLT(*) Handbook (https://www.swedishwood.com/publications/list_of_swedish_woods_publications/the-clt-handbook/) addresses the carbon emissions of CLT per component: Figure 1.3 in the book shows that ~15% of the carbon emission of the CLT-building(**) in the production phase comes from “plastic and glue” – this is surely a large percentage, but the overall emission of the CLT-building is still ~40% lower than the concrete-framed building. Figure 1.4 expands the emission analysis to the entire lifecycle: CLT-building still has an advantage over concrete-framed building (***).
(*) CLT = cross laminated timber. It is not exactly “glulam”, but it is also made of glued timber boards.
(**) “CLT surface units” in the figure.
(***) Note that the values in this figure is not directly comparable with Table 1 in the blog article, because the account methods are different.